- A turbocharger is made up of two main sections: the turbine and the compressor. The turbine consists of the turbine wheel (1) and the turbine housing (2). It is the job of the turbine housing to guide the exhaust gas (3) into the turbine wheel.
- Turbo technology evolved rapidly during the war years. The full strength of blowers was certainly tested during World War II. And as you can imagine, the B-17 and the B-29 bombers, along with the.
The turbocharger is the single most significant engine component or add-on device for increasing horsepower in the internal combustion engine. There may be arguments to the contrary, but I make this statement with good reason. To fully understand this concept, it’s important to look at an engine in a very fundamental way.
How a Turbo System is made up. The components that make up a typical turbocharger system are: The air filter (not shown) through which ambient air passes before entering the compressor (1); The air is then compressed which raises the air’s density (mass / unit volume) (2) Many turbocharged engines have a charge air cooler (aka intercooler) (3) that cools the compressed air to further.
This Tech Tip is From the Full Book, TURBO: REAL WORLD HIGH-PERFORMANCE TURBOCHARGER SYSTEMS. For a comprehensive guide on this entire subject you can visit this link:
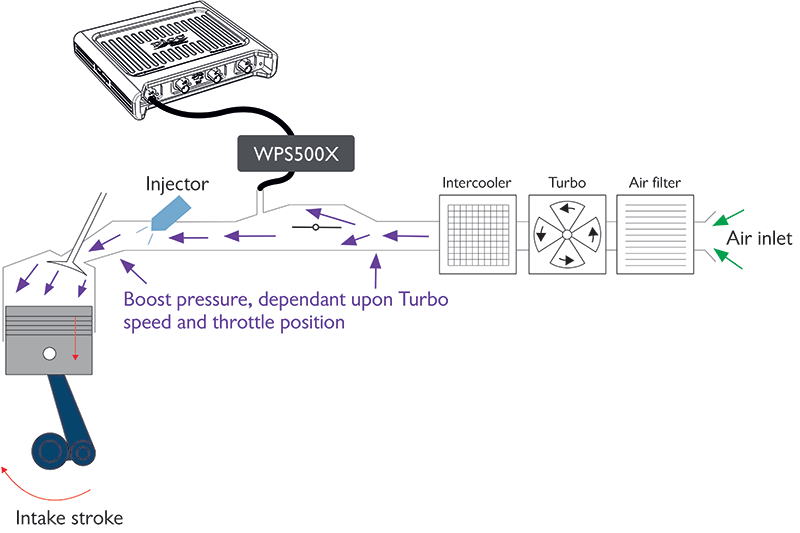
LEARN MORE ABOUT THIS BOOK HERE
SHARE THIS ARTICLE: Please feel free to share this article on Facebook, in Forums, or with any Clubs you participate in. You can copy and paste this link to share: https://musclecardiy.com/performance/introduction-turbochargers-questions-answered/
An engine is first an air pump. But its primary purpose is a device that converts the combustion of various types of fuel into mechanical energy so that it may be used in a constructive and controlled manner. This conversion of thermal energy into mechanical energy is performed by several simple machines contained within the engine. The specifics of this series of simple machines within the engine vary by design and constitute the differences between particular engine types. Clearly some designs are better than others, but that is a separate issue. For non-turbocharged engines, “breathing” is a challenge. This is evident in the NHRA Pro Stock ranks, a racing class where turbos are not allowed. These engines arguably represent the ultimate in power producers among all engines that do not use a forced air induction system. Most advanced engine builders agree with the fact that engine design considerations within the short block relative to improving airflow are basically maxed-out. For Pro Stock competitors, the trade secrets for increasing airflow lie within the intake manifold and cylinder head design. That’s where all of the work and technology is applied in a naturally aspirated engine simply because these engines lack forced air induction.
The Banks Twin Turbo Small Block Chevy is perhaps the world’s most recognizable twin-turbocharged engine assembly. (Courtesy Gale Banks Engineering)
However, the fundamental aspect of an engine as an air pump is the key to horsepower development. The more air that an engine can pump, the more horsepower it can develop. It’s important to always remember however, that more air doesn’t make horsepower—more fuel does. As a general rule, getting more fuel into an engine usually isn’t the problem; getting enough air to burn it is. Any engine design can produce more power with the addition of a turbocharger, but only within the limits of its ability to cope with the stresses of producing additional horsepower.
Understanding How a Turbo System Works
Horsepower is only produced by the combustion of fuel. Therefore, the more fuel that is burned, the greater the horsepower produced. But the fuel can only be productively burned if it is burned in the engine’s cylinders and there has to be enough air present to do this. We live in an atmosphere that, at sea level, contains 14.7 pounds per square inch (psi) of pressure. This means that an engine’s ability to breath is limited by the vacuum, or suction, created by the intake stroke of the engine’s piston, fed by a maximum pressure of approximately 15 psi. So, even in the most miraculously efficient engine design, there can only be a maximum pressure differential between atmosphere and the intake valve of about 15 psi absolute pressure. And since few of us live and drive on the beach, we have even less than 15 psi to work with. As you rise in altitude atmospheric pressure drops.
Now comes the turbocharger. In the most basic definition, a turbocharger is a device that drives more air into an engine. The 15 psi of absolute pressure is now boosted to something greater, and thus more air is forced into the engine’s cylinders. Now the fuel delivery rate can be increased and more horsepower will be developed. In many cases, a turbocharger can deliver enough additional airflow such that the same size engine can easily produce 100 percent or more horsepower than in its naturally aspirated, or nonboosted, state.
This illustration shows a simple aftercooled single turbo system. Let’s follow the intake airflow to see how it works. (Courtesy Honeywell Turbo Technologies) A. After the intake air passes through the air filter it is routed to the turbocharger’s compressor inlet (1). B. The air is then compressed, which increases its density—or amount of mass per unit of volume—by the turbo’s compressor section, and is discharged through a boost tube (2). C. Most of today’s turbo systems use an aftercooler, also known as an intercooler or charge-air cooler, to receive the hot, boosted air as it leaves the compressor. The cooler removes the excess heat, which allows for further air density improvements while also cooling the intake air charge (3). D. Another boost tube then routes the air from the cooler’s discharge side to the engine’s intake manifold where the air is routed to the intake valves and it enters each cylinder (4). E. An increased fuel injection charge, based upon the now more dense air charge, is introduced and combustion occurs. After combustion, the exhaust passes through the exhaust valve and into the exhaust manifold (5). F. The exhaust manifold routes the exhaust gas energy in the form of heat and pressure into the turbine stage of the turbocharger (6). G. The turbine housing causes backpressure. This backpressure and heat expand against the turbine wheel blades causing the turbine wheel and shaft to spin, which in turn drives the compressor wheel on the intake side of the system. The remaining exhaust then leaves the turbine stage and enters the exhaust system (7).
Turbocharger, supercharger, turbosupercharger, and blower, among other names, are technically correct when referring to any of several different types of devices that perform the principal function in a forced air induction intake system on an internal combustion engine. But among enthusiasts, power wizards, bench racers, and even a few well-read squirrels, supercharger and blower are used synonymously to refer to devices that increase the intake pressure and are powered directly off of the engine’s crankshaft. This book principally concerns itself with the turbocharger, which derives its power from exhaust gas energy through the use of a gas turbine. This difference in technology is the basis for great debate among the performance community about the superiority of superchargers versus turbos. Historically, each device has areas of supremacy over the other, but those areas of application superiority are beginning to narrow, as this book will outline in forthcoming chapters.
Turbochargers reign supreme for one simple reason: The turbocharger is the most efficient of all the economically applied forced air induction devices. Unlike the supercharger, which takes power directly off of the crankshaft to drive its compressor, the turbocharger utilizes energy present in the exhaust gas that would otherwise be wasted by simply pumping it into the atmosphere. But even though a turbo uses exhaust gas energy that is commonly referred to as waste energy, there is a cost of using this energy that comes from the increased backpressure formed in the exhaust. Individuals applying turbos to engines frequently overlook the concept of “pumping losses.” Well, there is no free lunch. The relationship between backpressure and turbos are an important concept that must be balanced and is dealt with in greater depth when we get into matching a turbo to a specific engine (Chapter 3).
A Brief History
Turbocharger Tutorial Vector
According to most, the turbocharger is credited to a Swiss engineer named Alfred Buechi. It was Buechi’s application for patent of a compound motor in 1905 that started the ball rolling. This patent did not describe the turbocharger as it is known today, but rather an axial flow turbine and compressor that shared a common shaft with the engine they were applied to. This early design was not a commercial success but gave rise to further developments in the area of use of gas turbines and compressors aiding engine performance.
Alfred Buechi, inventor of the turbocharger. (Courtesy ABB Turbo Systems LTD. Baden, Switzerland)
It wasn’t until 1916 when Professor Auguste C.E. Rateau, the French inventor of a then well-known steam turbine, applied for a patent. The patent, however, was not published until 1921. Meanwhile, during late 1917, the National Advisory Committee for Aeronautics, to consider rapid development of the turbocharger to support the war effort, approached Sanford A. Moss of General Electric (there is no known collaboration between Rateau and Moss). Research was carried out at Pikes Peak in Colorado, approximately 4,250 meters above sea level. At that time there were altitude test chambers for aircraft engines, but not for an engine, a turbocharger, a propeller, and all the accessories. Tests proved that sea level power could successfully be attained at altitude with a turbo, making turbocharging a solution for military aircraft to achieve higher speeds and altitudes.
The end of World War I slowed turbo development, but it provided time to consider design and testing issues. General Electric continued its research. During the World War II, Moss’s turbochargers were used extensively in aircraft and bombers like the well-known B-17 Flying Fortress. The turbocharger certainly had its contribution to the war effort and made a significant contribution to the Allied victory.
As with so many products and ideas, necessity is the mother of invention. The turbocharger is no exception. If engines are to be applied to a variety of uses, breathing is fundamental to their success. The internal combustion engine has reshaped our world and the turbocharger has helped the engine accomplish that task.
The Rise to Popularity
The postwar era saw little use of turbochargers at first, but gradually the turbocharger became a sought after component as postwar expansion gave rise to great economical development. Commercial manufacturers employing diesel engines, such as the Caterpillar Tractor Company realized that there was a constant increasing need for more power from their machinery. Without turbochargers, achieving power objectives would drive up engine size and cost. Certainly, a manufacturer who had twice the engine power versus a competitor would have a significant advantage.
The current Schwitzer-BorgWarner model S3 on the left has a slightly higher airflow range than the older Schwitzer model made in the ’60s for Cummins Engine Company. While the intended applications are different, it’s easy to see the dramatic design differences that have come about from computer-aided designs, improved materials, and manufacturing processes. (Courtesy Diesel Injection Service Company, Inc.)
The 1950s and ’60s saw a significant growth in turbo use. The primary growth took place in commercial diesel engines. This spawned the rapid growth of companies that would specialize in the development of turbocharging technology worldwide. The postwar economic expansion spawned rapid commercial growth, and more uses for turbochargers brought about expanded research and development from commercial competitors, which bring us to the modern era of turbo technology.
As turbo reliability and designs improved, the applications for turbos expanded. The muscle car era of the ’60s saw Detroit attempting to turbocharge a few gasoline automobiles such as the Chevy Corvair.
Monza and the Oldsmobile Jetfire. These applications were problematic at best. There are many opinions as to why turbochargers failed on this first attempt with production automobiles, but I maintain that the reasons are many.
The available engines were not designed to be turbocharged and there wasn’t a wealth of turbo application experience in the industry as it related to passenger cars. The turbo design that had to be considered for use on a carbureted engine had problems with durability and getting the fuel flow correct was problematic. Consumers could get all the horsepower they wanted with larger-displacement engines, high compression ratios, high-lift cams, and more. The Baby Boomer gearheads weren’t ready for turbos. Besides, with gasoline at only 20 cents a gallon, who cared that you only got 6–8 mpg. Mileage concerns were for wimps! Wanna go fast? Cubic inches could get you there.
When the Arab oil embargo of the 1970s hit, spurred on by the clean-air act of 1977, Detroit began to focus on fuel efficiency and lower exhaust emissions. Smaller engines began showing up to burn less fuel and give consumers greater economy. But Americans still wanted their horsepower.
In the 1980s, Detroit returned to turbocharging as a way to achieve higher horsepower and performance for the now smaller engine packages. While some applications became famous such as the Buick Grand National, there were still problems in turbo land. The high exhaust temperatures seen in gasoline engines and poor maintenance by consumers weren’t the only challenges.
The technical problems associated with turbocharging by the average doit- yourselfer mostly centered on the issue of whether to “pull through” or “blow through” the carburetor. If you used a blow-through method you would maintain optimum placement of the fuel distribution from the carburetor, through the manifold. But the problems associated with this method include boost enrichment of the fuel flow, because a carburetor senses airflow, not air density.
The pull-through method was favored by most application engineers because the turbo’s compressor was located downstream of the carburetor. This position in the system meant that the carburetor could sense the increase in volumetric airflow that was feeding the compressor prior to the turbo increasing the air density by building boost. In this way a separate boost-enrichement system for fuel to increase during boost conditions was not necessary, the carburetor mostly took care of it. The pull-through method, however, still had its problems because the air and gas mixture was run through the turbo’s compressor. This meant that the compressor cover must be tightly sealed with gasket sealer and/or an O-ring to the bearing housing so that raw gasoline could not leak out since it was now pressurized. The compressor would also act as a centrifuge to separate the gas from the air since the air and fuel dropets have different specific gravities. In addition, since the compressor was routed downstream of the carburetor’s throttle plate, the compressor was subjected to engine vacuum during light loads, or partial-throttle, noboost conditions. Under such conditions, engine oil would be literally sucked right out of the turbo and into the engine. In order to solve that problem, positive type carbon face oil seals were used on compressors, but they were notorious for short life. Further, the drag on the turbine shaft caused by the positive type seals consumed a portion of the turbine’s power, thereby lowering the available energy to drive the compressor.
The rapid introduction of electronic fuel injection to gas engines in the ’80s was the great enabler. Electronic fuel injection meant no carburetor and no carburetor meant easier turbo retrofits! Aftermarket turbo systems could now be more easily installed. This led to a dramatic increase in interest in aftermarket turbo setups.
Understanding the Turbocharger
Understanding how a turbocharger works is fundamental to the successful application of a turbo and to matching it to an engine correctly. Perhaps the best way to understand how a turbo works is to break it down into its primary sections. This is done in great detail in Chapter 2. But as a preview, the turbocharger simplified has basically three areas of design: the turbine, the compressor, and the bearing system that ties the compressor and turbine ends together. Each of these areas is interdependent on the other for overall turbocharger effectiveness, but they are each developed as discrete machines. Turbocharger manufacturers commonly separate the design engineering teams into areas of specialization where you will find turbine development specialists, compressor specialists, and mechanical design specialists for bearing and support systems.
The Turbine
Let’s begin with the turbine. A turbocharger obtains its energy in the form of heat and pressure from the engine’s exhaust. Any given turbine has a designed set of parameters that dictate what amount of exhaust flow it is compatible with. A properly applied turbine will actually raise the backpressure in an engine’s exhaust system, which will, in turn, raise the pressure to drive the turbine and increase the heat in the exhaust system.
The turbine has two principal components; the turbine wheel and the turbine housing. The exhaust from the engine is routed to the turbine through the exhaust manifold and enters the turbine inlet. The exhaust gas fills the turbine housing and creates a static pressure by way of a volute shaped turbine housing that feeds the turbine wheel at its tip or the inducer area of the turbine wheel. Since high pressure always seeks low pressure, the exhaust gas flows through the turbine wheel and the high-pressure gas expands as it travels through the turbine wheel. This exhaust gas expansion is what drives the turbine wheel in rotation inside the turbine housing.
The turbine is the machine that extracts the energy present in the form of heat and pressure from the exhaust and converts it to mechanical energy via the turbine wheel’s rotation. Therefore, turbine design and efficiency is critical to overall turbocharger effectiveness as it relates to engine performance. Even more important than subtle design enhancements within the turbine is a properly sized turbine to correctly “see” the engine’s exhaust.
This is a cutaway photo of a turbocharger showing the major sections of turbo design. (Courtesy Honeywell Turbo Technologies)
The turbine section of a turbocharger consists primarily of the turbine wheel and turbine housing. This 120-degree cutaway section illustrates the turbine wheel position relative to the turbine housing. (Courtesy Diesel Injection Service Company, Inc.)
The household garden hose is a simple analogy to help understand optimized flow matching. If you let the hose run with the end unrestricted it flows out in high volume and will typically run out 3 to 6 feet from the hose end. Now begin to restrict the hose end a little at a time and you find the pressure rising and the water sprays out farther and farther. It may spray as far as 30 to 40 feet. But if you continue to cover more and more of the hose, at some point you see a point of diminishing return. The water spray distance begins to drop off because you have restricted flow too much and all you have done is caused excessive backpressure beyond what was optimized for maximum flow pressure and volume.
A turbine is sized for a given engine’s exhaust gas flow in much the same way. But since an engine operates at a variety of engine speeds, the challenges are more complex than the constant flow through the hose. For example, a given engine that is used in a street driven vehicle and reaches engine speeds of only 5,000 to 6,500 rpm may require one size turbine.
The same engine designed to perform in a racing environment and operate at engine speeds of 7,000 to 9,000 rpm will need a completely different sized turbine. It’s extremely important to understand the turbine-to-enginesize relationship because all too often turbos are mismatched to engines based upon the premise that smaller turbines raise boost pressure. While that may be true, the point of diminishing return is often met or exceeded and a turbine housing that is too small is now robbing horsepower due to turbine choke and what is known as pumping losses (excessive backpressure). This will be discussed in greater detail in Chapter 3. But for now it is only important to understand this flow relationship and how a turbine can be sized too small as well as too large.
The Compressor
As the turbine wheel rotates from the hot high-pressure exhaust gas expanding through it, the wheel is connected to a shaft that has a compressor isolated at the opposite end. The compressor performs a job that is just the opposite of the turbine. The compressor’s job is to gather fresh air and raise its pressure before it enters the engine.
The compressor also has two primary components: the compressor wheel and the compressor cover. The compressor wheel is a radialtype compressor, meaning that as the air enters the leading edge of the wheel called the inducer, it is accelerated and turns 90 degrees and exits the compressor wheel perpendicular to the turbine shaft that drives the compressor wheel. The energy extracted from the turbine is used for work to spin the compressor wheel that pulls air into the wheel and then compresses it.
The compressor section of a turbocharger consists primarily of the compressor wheel and compressor cover. (Courtesy Diesel Injection Service Company, Inc.)
As the air leaves the compressor wheel it enters the portion of the compressor called the diffuser. The diffuser converts the air into static pressure and fills the compressor cover. The compressed air leaves the compressor cover and is routed by way of a boost tube directly into the engine or to an aftercooler, and then enters the engine. The compressor has two functions. The first is to raise the intake pressure to aid the engine in its ability to breathe. Higher intake pressures allow for more complete filling of the engine cylinders with air. The other function is to increase air density. An engine reacts favorably to air density more than boost pressure, and air density is the primary objective of higher boost pressures. However, boost pressure is a critical element as well and is directly responsible for the increase in volumetric efficiency, a concept explained in greater detail in Chapter 3.
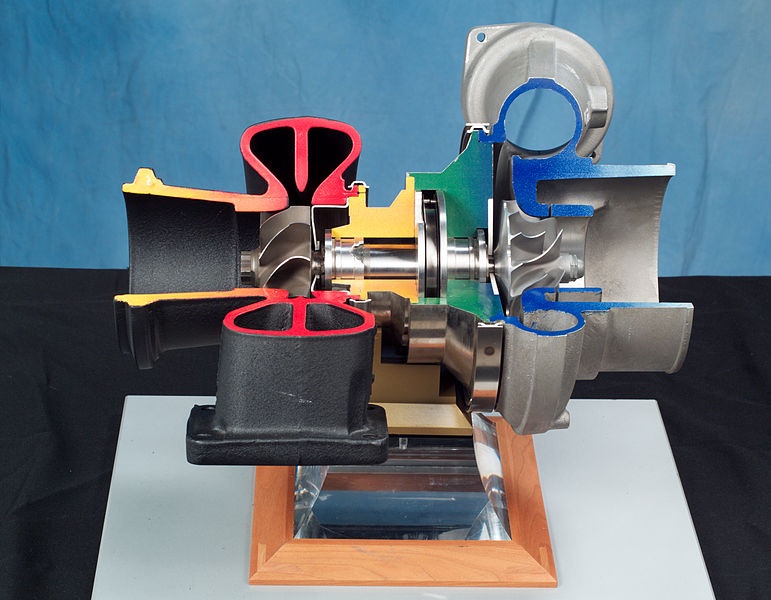
An engine has a fixed amount of volumetric capacity or displacement. Therefore the air pressure and density must be increased so that the engine will flow a higher mass of air, which allows for a higher mass of fuel to be introduced. The boost pressure level for a given compressor is highly dependent upon that compressor’s ability to efficiently compress the air without adding excess heat. The concept of density is simply stated as follows: Density = mass per unit volume. Boost pressure will rise as a function of two variables: air temperature and air density. It is the air density increase that allows more fuel to be burned and therefore, more horsepower to be developed.
If two sealed cans were both fitted with pressure gauges and one was heated, its pressure gauge would rise like boost pressure. However, there would be no higher air density in the heated can, just more heat and pressure. The excessive heat is not desirable, but is an example of what happens when the incorrect compressor is installed onto an engine. If an engine requires more air than the compressor can efficiently compress, the air gets extremely hot and engine problems, such as detonation, are more likely to occur.
Proper sizing of the compressor is just as critical to maximizing engine performance as it is with the turbine. Each compressor design is trimmed to flow the amount of air compatible to an engine’s air requirement based upon that engine’s cubic inch displacement, volumetric efficiency, and operating RPM. A compressor that’s too small restricts the engine and limits horsepower. Likewise, a compressor that’s too large requires more power to spin than the turbine is capable of developing and thus will not spin fast enough to supply the airflow and pressure that compressor would otherwise be capable of developing. Thus there is a power balance that clearly becomes obvious and important between the compressor and turbine ends of the turbocharger.
The Bearing System
The turbine shaft is supported by a bearing system that links the turbine to the compressor. The bearing system is housed inside of a bearing housing that contains many design features critical to proper turbo operation. The bearing system has a tough job. It must support the turbine and compressor wheels as they rotate to speeds in excess of 100,000 rpm while surviving various gyrations induced by engine pulses that vary with engine speed and power output. In addition, the bearing system must also absorb not just radial loads but thrust, or axial loads as well. When turbine pressure exceeds compressor pressure or vise-versa, there is thrust on the bearing system. Pressure differentials that become too severe will fail a good turbocharger.
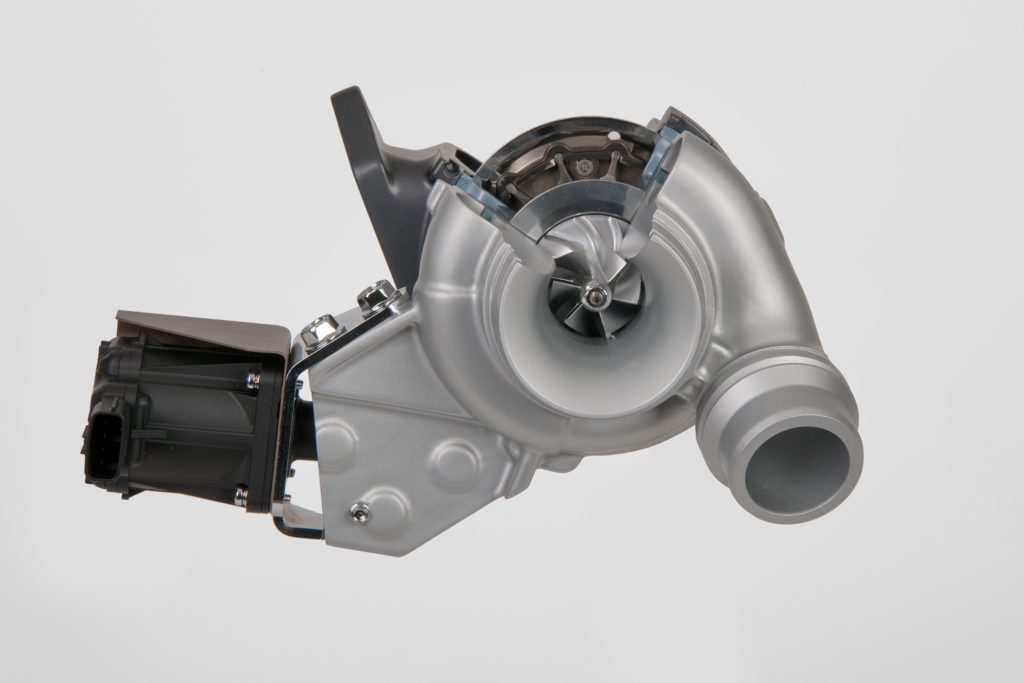
The turbocharger bearing system is contained within the bearing housing. The bearing housing supports the turbine housing and compressor cover, while the turbine shaft and bearings support the rotational dynamics of the turbine and compressor wheels. Most turbocharger bearing systems are bronze type journal bearings that have oil passages to feed the hydrodynamic lubrication system, while many late-model high-performance turbos utilize specially designed ball-bearing cartridges.
While there are many types of bearing systems they all must do this job while providing a minimum of drag or frictional loss of energy extracted from the turbine to power the compressor. The bearing housing is also home to many more design features that include oil control, gas seals, heat dams, and, in some designs, water jackets to keep extremely high exhaust temperatures away from the lubricating oil.
Where and Why Turbos are Used Today
The word “turbo” has been widely used to describe everything from computer software to shoes. Marketers use the word “turbo” to convey power, speed, and a superior level of performance. Those of us who work in the turbo industry see humor when somebody flips a switch labeled “turbo” and a sudden power surge is produced. It doesn’t work that way. While the term has been glamorized by this over use, there is good reason for it. The turbocharger has successfully worked itself into just about every engine application, with the exception of NHRA Top-Fuel Dragsters and Funny Cars, where they are banned outright.
The turbocharger’s development was accelerated due to the need that aircraft had to achieve high horsepower levels in the thinner air seen at high altitude. Turbine speed increases about 2 percent per 1,000- foot increase in altitude. Both aircraft and commercial engine equipment benefit from this natural altitude compensation characteristic in turbochargers. The jet engine made the piston engine obsolete for most commercial passenger planes today, but turbos are still applied to lower-cost small aircraft that use piston engines.
Today’s diesel engine, whether a large commercial diesel truck, earthmover, or consumer diesel pickup, is turbocharged. The reasons are both for power and emissions. As Gale Banks says, “A diesel engine is just not complete until it is turbocharged.” The high-BTU (British thermal unit) property of diesel fuel makes it virtually impossible for a naturally aspirated diesel engine to breathe enough air on its own to burn all of the fuel completely. Gasoline contains about 125,000 BTU per gallon, while diesel contains about 138,000. I don’t believe there has been an on-highway commercial diesel engine (class 4 through 8 trucks) produced without a turbocharger for nearly 30 years.
The racing community discovered turbos many years ago. There has been a steady evolution of more and more classes of racing that accept turbochargers. The sport compact class in NHRA is one of the most recent examples of this movement. For many years turbos were seen almost exclusively in the Indy car circuit, where high budgets were the norm. For most other classes of racing the sportsman populated the ranks, and turbos were exotic and expensive and viewed as an unfair advantage by most average racers. That is now changing. While turbos are still expensive, the real expense of the pioneers was in trial and error to obtain the correct and optimized match in a world of minimal hardware options. That’s no longer the case, as turbos are widely available in a variety of sizes to match virtually any engine design.
Today the sport and pleasure market is completely covered by turbos. Whether it’s a sport car, recreational vehicle, or a boat, turbos add driving pleasure. The turbocharger will return to several more automobiles than are currently available very soon, and for one simple reason— efficiency won’t be denied. Higher fuel prices are probably here to stay, as is the increasing demand for fuel economy. It’s cheaper for the automakers to manufacture a 6-cylinder turbocharged engine than a comparably powered naturally aspirated V-8. The smaller engines fit into smaller vehicles and fuel economy gains are seen from both the smaller engines, as well as the smaller and lighter vehicles where they are used.
Types of Turbocharger Systems
The turbocharger can be applied in a variety of ways. The intended use of the vehicle tends to dictate what type of turbo system is best applied to the engine. The most common system is the single turbo matched to a specific RPM and horsepower point.
The twin turbo set-up is a highly specialized system where two identically sized turbos are matched to approximately one half of an engine’s need for airflow. A typical application is the V-8 where 4-cylinders feed each turbo. This approach is typically used for racing where rapid acceleration is needed and the smaller wheels inside each turbo spool up faster than one larger unit would. It is also used in the street rod market for the design beauty that comes from the more sophisticated, symmetrical look. This is commonly found in open-air engine bays where builders are as much showing the engine as they are the rest of the car.
Note: There are important considerations to the twin turbo concept given today’s technology as will be discussed more in Chapter 6, Designing a Turbo System.
Multi-stage (or compound) turbo systems are a very exotic method of turbocharging and are usually reserved for extreme applications such as tractor pulling or similar high-performance applications. However, Caterpillar manufactures a 550-hp truck engine that uses two turbos staged together to reach higher boost levels and air density than could be durably achieved using only one turbo. Using a firststage, second-stage compressor allows for higher air density from the higher boost pressure without the overspeed condition that would be required to achieve the same total pressure ratio from a single turbo. This type of system also contains what the application engineers refer to as “all kinds of map width,” a concept that refers to a compressor’s mapped flow range potential discussed in Chapter 3. It has essentially the turbo surge margin of the small turbo and the choke flow output of the larger turbo.
This 1999 Case-IH MX150 uses a fixedgeometry, or free-float type turbine housing, which means no wastegate or other type of variable turbine geometry. This type of turbo system is the most commonly found type in use today. The engine is rated at 130 hp, with 5.9 liters of displacement and a Holset brand H1 model turbocharger. Most agricultural tractors, from brands such as John Deere, Case-IH, Massey Ferguson, etc., rated over 100 hp use a turbocharger. All of today’s on-highway diesels use a turbocharger because they have to in order to meet emissions regulations.
Gale Banks Engineering makes a marine twin-turbo kit for the 454-ci big-block Chevy that produces nearly 900 hp. Note the water-cooled exhaust manifolds, and pull-through carburetor arrangement.
Manny Cruz of the Bronx, New York, uses a pair of Garrett GT42 turbos on his 3.0-liter V-6 Mercury Cougar, which races in the Pro Rear Wheel Drive Sport Compact class. The compressor outlet from each turbo is routed forward where they enter the aluminum cased water-to-air aftercooler (1), and from there, the aftercooler combines the air from both turbos into a single duct boost tube (2) that enters the engine’s intake air plenum (3).
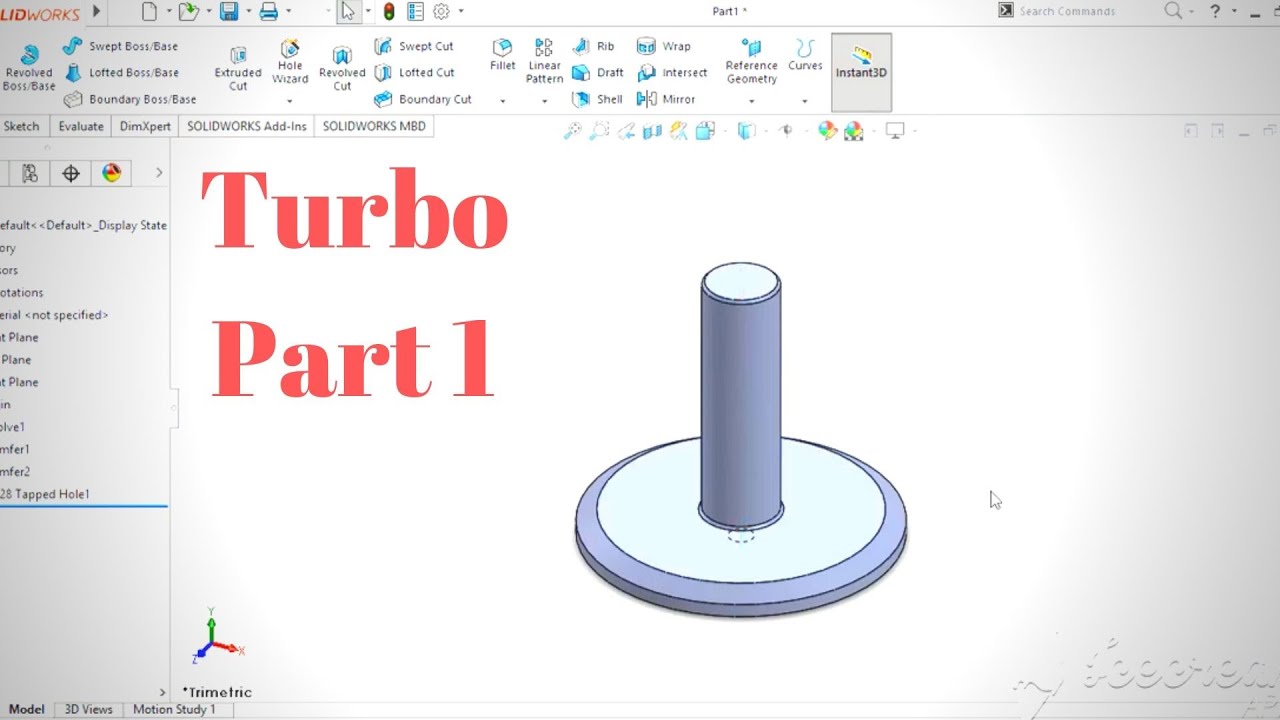
Solidworks Turbocharger Tutorial
This C15 Caterpillar engine is popular among many line-haul fleets and owner-operators requiring high horsepower. This 15-liter engine uses a compound turbo setup, which is commonly, but incorrectly, called a twin-turbo system. Twins are two identical turbos where each turbo is matched to flow one-half of the engine’s air-mass demand and each turbo feeds the engine directly. In a compound turbo system, one turbo feeds the next for a compound effect to create a second stage of compression. The engine comes in ratings ranging from 435 hp with 1,550 ft-lbs of torque to 625 hp with 2,050 ft-lbs of torque. While these ratings may not sound astonishingly grand to high-horsepower enthusiasts, the engineering feat is that these commercial engines can produce this output all day long, and not require engine rebuilding for 500,000 to 1,000,000 miles!
The Garrett Model GTP38 as shown was used on the 7.3-liter Ford Powerstroke diesel engine in heavy-duty pickups. This turbocharger used a pedestal mount and employed a wastegate assembly that allowed improved low-end response. (Courtesy Diesel Injection Service Company, Inc.)
The robust design of the diesel engine can withstand this level of power output and commercial diesel engines are sold and priced by horsepower. Independent truckers like higher horsepower because they never know what their next load will be, or whether or not they’ll be hauling up through the mountains. In a typical staged turbo setup, the exhaust leaves the first turbo and enters the second turbo. Similarly, the compressor outlet of the first stage is fed into the intake of the second stage turbo, much like a twostage air compressor in a typical workshop. Tractor pullers have used these types of two, three, and even four stage turbo systems to build incredibly high pressures and achieve power levels that the engines could not withstand in a work environment.
In fact, many times they don’t even last through one full-pull! The pressures seen in these extreme applications frequently reach over 100 psi, while still others have achieved boost levels as high as 200 psi!
Another variation to the single turbo system uses a wastegate. The wastegate is located either inside the turbine housing or as an add-on device that is installed in the exhaust manifolding upstream of the turbine. The wastegate is applied to an application such as an automobile where turbo boost is desired at lower engine speeds, so a small turbine is used. Yet the engine’s natural operating RPM is over a wide range and will frequently achieve an exhaust flow volume that would over-speed the turbine and choke the engine. The wastegate allows a simple bypass whereby a valve opens at a preset point and allows the excess exhaust energy to bleed off. This allows more exhaust flow past the turbine instead of choking the engine, while also allowing boost to be controlled, as the power driving the compressor is regulated.
Wastegates are a vital component in any gasoline engine due to the wide RPM range of operation. Recent history has seen wastegates also used on several commercial and consumer diesels. The wastegate is the simplest form of variable geometry. They are widely used and have good acceptability and durability in most applications, but there is a superior concept.
Introduction to Variable Geometry Turbochargers
Variable geometry is the design of a turbine to vary its geometric size and therefore its capacity for swallowing exhaust gas flow. Variable geometry, or VG, turbos are a much more sophisticated form of turbocharging. Today’s high-speed diesels and gasoline automobiles demand higher efficiency. A wastegate does just what its name implies it wastes energy that could otherwise be put to good use as energy to drive the compressor. There were working VG turbochargers as far back as the mid ’80s, but manufacturing costs and fuel mapping technology meant VG wasn’t quite ready for primetime. In a VG turbine, a series of vanes is positioned around the turbine housing in such a way as to throttle the exhaust gas volume and pressure as it enters the turbine wheel. It works much like the garden hose spray nozzle end where you can vary the opening nozzle anywhere from nearly closed to wide open. This allows the same turbine to act as a small turbine at low engine speeds and as a larger turbine at higher engine speeds. Unlike a wastegate, with VG all of the energy is transmitted to the turbine wheel to build power.
Variable geometry turbines offer a slight efficiency gain over the same wheel and turbine housing design without VG. This is the result of the vanes being positioned at the most optimum angle of incidence relative to the turbine wheel. Tests have shown about a two-percent turbine efficiency gain with VG.
Check out the inner workings of a variable geometry turbine with the turbine housing striped away. The series of vanes are positioned on a unison ring that rotates to open and close the vanes, thereby adjusting the amount of exhaust energy allowed to reach the turbine wheel. (Courtesy Honeywell Turbo Technologies)
Turbocharger Tutorial Minecraft
This is the Cummins ISX engine in a Kenworth truck application. This late-model, emissions-certified commercial diesel engine uses a Holset variable geometry turbocharger to help create outputs from 385 hp and 1,550 ft-lbs peak torque to 600 hp with 1,850 ft-lbs, both at 1,200 rpm.
Commercial applications of VG turbos are now in the market. Detroit Diesel Corporation uses VG in their current Series-60 commercial diesel model engines. The latest GM Duramax, Dodge/cummins, and Ford Power Stroke diesels in pickups and midrange diesel trucks are also using VG turbines. While these applications are using VG for efficiency and emissions purposes, the fact that VG is now into the mainstream means it’s only a matter of time before its use changes the face of performance turbocharging in the automotive aftermarket.
To date there have been very few gasoline engine applications. The VG used on most production engines is presently applied to diesel. Diesel runs significantly lower exhaust temperatures than gasoline. The current VG designs are not very compatible with gasoline. But applying VG to gasoline isn’t as much about feasibility as it is affordability. Current VG components use parts with high nickel content. The use of cobalt would likely be required to be successful and durable for gasoline but would significantly drive up the manufacturing cost. Alternative designs for gasoline engines are being evaluated to find lower cost methods of durably applying VG. One such thought is a design where instead of a series of vanes, an axially sliding orifice progressively increases and decreases the port entry from the turbine housing to the turbine wheel blade tips. Holset brand turbochargers use this type of VG that’s now in production on the 2007 Cummins diesel in Dodge trucks.
The Future
The future for turbochargers is very dynamic. Today, carburetors are a distant memory on the street and fuel injection reigns supreme. Emissions, engine durability, and turbo compatibility are significantly enhanced through the use of electronic fuel injection. Gone are the large-bore torque monsters of the ’60s and ’70s. Today’s highly refined engines are smaller and making more horsepower per cubic inch than ever before.
Turbochargers are also so much more refined. A wide selection of turbo models and flow ranges are available. Fixed geometry, wastegated turbos, and even full variable geometry is now in production on many applications. Advanced materials and designs solve several problems of the past, and getting an engine to work with its turbo system is now so possible for virtually anyone because of the available system components and integrated electronic engine management and support systems.
It used to be that large turbos required for small engines to build high horsepower suffered from excessive system lag at vehicle launch. Today, with higher aerodynamic efficiencies, ball bearings, sophisticated fuel mapping, and twostep/ anti-lag strategies, lag is simply another resolvable tuning issue. Over the next few years the automotive manufacturers will use turbochargers more and more. The cost of a turbocharged V-6 is less than the cost to produce a naturally aspirated V-8 with the same horsepower, and the cost of a turbocharged 4-cylinder is less than that of a V-6. Diesels will continue to command more market share and every diesel will have a turbocharger because they have to in order to meet emissions standards.
Holset Brand turbos use a slightly different design in their Variable Geometry. Instead of the vanes themselves actuated by a unison ring, the vanes remain in a fixed and optimum aerodynamic position. A shroud plate moves over the fixed position vanes to progressively open and close the orifice leading from the turbine housing volute through the vanes to choke the turbine for low-end performance or open up the swallowing capacity for high RPM operation. (Courtesy Cummins Turbo Technologies)
The Garrett LST (Low-Speed Turbo) uses two separate compressor wheels, driven by a single turbine. They are positioned in a first-stage, second-stage arrangement to achieve higher boost pressures from lower shaft speeds. This reduces compressor wheel stress for longer in-service life. The first-stage compressor wheel is aluminum while the second stage is made from titanium that will better withstand the high heat from the first-stage during second-stage compression. (Courtesy Honeywell Turbo Technologies)
The new Garrett SST (Single-Sequential Turbo) uses two Siamesed compressor wheels formed into one wheel casting, driven by a common turbine shaft. Note how each compressor wheel discharges into a common volute in the compressor cover. (Courtesy Honeywell Turbo Technologies)
While today’s high-performance aftermarket products are very advanced and allow for superior turbo tuning, wastegate control is still the dominate means of tuning a turbocharged engine to provide high amounts of low-end torque, while still allowing the engines to rev to their potential. I remember Steve Arnold, Honeywell Turbo Technologies’ director of innovation & new concepts, referring to the wastegate over 25 years ago as an “aptly named device.” Full variable geometry is now making its way into production vehicles. Most of the new commercial and consumer diesels coming out are equipped with full variable geometry turbochargers. While the present use of variable geometry is mostly applied to diesel applications, it’s only a matter of time before continued refinements in materials and design become mainstream on gasoline automobiles.
By the year 2010 new design concepts will begin production such as Garrett’s new Low Speed Turbo (LST). The LST is a creative concept that uses two compressors staged in series, or compound compressors, designed integral into one compressor cover driven by a common turbine. The LST is capable of comfortably achieving higher pressures without over-speed to the turbo and limiting its durability. In fact pressure ratios of 6:1 are well within this design concept, and the flow range of the compressor, surge to choke is nearly double that of its similarly sized single-stage wheel. The LST applied with a variable geometry turbine will make for some interesting aftermarket applications.
Another new design that will likely see production in 2010 is the Garrett Single Sequential Turbo (SST). This design uses essentially two compressor wheels in one casting that share a common backwall. The two compressors flow in parallel. Their application is intended for high- BMEP (Brake Mean Effective Pressure) engines that have a wide speed range such as turbo diesels used in lightduty pickups, SUVs, and passenger cars. Due to the twin compressor wheels flowing in parallel, the overall rotor mass and resultant moment of inertia is reduced by around 40 percent. This directly effects turbo acceleration. The choke to surge is about a 3:1 ratio and the turbine efficiency gains from this compressor design are in the range of 10 percentage points, which is a huge gain.
The automotive aftermarket will always want its horsepower. The turbocharger of today is a high-tech engine component that possesses a great amount of sex appeal and gearhead attraction. The turbochargers of tomorrow will continue the trend of even higher sophistication and increased efficiency.
Written by Jay K. Miller and Posted with Permission of CarTechBooks
GET A DEAL ON THIS BOOK!
If you liked this article you will LOVE the full book. Click the button below and we will send you an exclusive deal on this book.
The Video Course teaches you everything about modern cars.

There are two main ways to get more power from a car's engine. The first(and until recently the most popular) is to increase the capacity of theengine. The second is to increase the amount of fuel/air mixture going into thecylinder.
Generally, the more fuel/air mixture going into the cylinders, the morepower the engine will produce. So part of the solution is to tune thecarburettor, cylinder head and manifolds to allow the engine to `breathe' morefreely, but there are limits to how much power can be extracted from an engineby these means while at the same time maintaining the engine's reliability andflexibility.
An alternative way of getting more fuel/air mixture into the cylinders iswith a turbocharger.
Unlike road cars, racing car engines do not have to compromise between power and flexibility, so they can be tuned for ultimate power at high revs because this is the speed range where they will spend most of their time when racing.With a turbocharged engine, this inevitably means running the engine at very high boost pressures as well as carrying out conventional tuning work.The most powerful turbocharged race engines can cope with boost pressures of 4-5 bar (60-70psi), whereas a turbo road car will run at a maximum of about 0.7 bar (10.5psi).What is a turbo?
A turbocharger is basically a pump driven by the exhaust gases passing outof the exhaust manifold. The unit consists of a wheel with vanes - the turbine- that fits inside a housing in the exhaust system. From this turbine a shortcentral drive shaft runs to a similar vaned wheel called the compressor thatfeeds into the engine's air intake.
from the engine, they spin the turbine, which in turn spins the drive shaftto turn the compressor. So, when the engine is running, the exhaust gases drivethe turbine which makes the compressor pump air into the engine.
A fixed amount of fuel is automatically sucked in with the air if the enginehas a carburettor. If the engine has fuel injection, the computer control unitis programmed to suit the boost pressures.
The faster the engine is running, or the larger the throttle opening orboth, the faster the turbocharger will spin. The faster the turbo spins, themore pressure, or boost it develops and the more air it forces into the engineto create more power.
Wastegates
Although the turbo is designed to pressurize the mixture going into theengine, too much pressure would be dangerous because it can lead to 'knocking'(pre-ignition) and put too much strain on the internal components of theengine. Therefore the maximum boost pressure that the turbocharger can producehas to be limited by a valve known as a wastegate.
The wastegate is a relief valve, located in the turbocharger, that opens tolet some of the exhaust gases bypass the turbine and flow directly into theexhaust system. If the boost pressure is getting too high, the wastegate isactivated by a pressure-sensitive actuator which senses the pressure beingproduced by the compressor.
Compressing the air causes problems of its own. When the air is compressedit heats up, which tends to make it expand. Because the purpose of the turbo isto get as much fuel/air mixture into the cylinder as possible, this hot airneeds to be cooled down.
To do this, most turbocharged cars are fitted with an intercooler. Thislooks like a small radiator, and cools the compressed air that leaves theturbocharger. As the air cools down, its volume shrinks, so the amount offuel/air mixture fed to the engine - and hence the power output -increases.
The turbo unit is plumbed in to the exhaust system as near to the engine aspossible. This helps to keep it compact and also helps prevent turbo lag. Ifthere was a long length of exhaust pipe between the engine and the turbo, therewould be a time delay between the accelerator being pressed down, the enginespeed increasing, and the turbo accelerating. The effect would be like havingan elastic throttle cable.
Therefore, the turbo is often bolted directly on to the exhaust manifold.The exhaust outlet is in the centre of the turbine housing and leads off to theexhaust pipe.
On the inlet side, the pressurized air leaves the compressor housing via alarge-bore pipe. This runs through the intercooler (if fitted), and then to theinlet manifold, or occasionally plenum chamber, where the fuel is added byinjection before the air enters the engine.
The high speeds at which the turbine can spin create lubrication and coolingproblems. In some turbochargers the turbine can spin at up to 200,000rpm, andthe hottest parts of the turbo will be at or near the temperature of theexhaust gas about 900°C.
Most turbo units have the central drive shaft bearing fed with oil from theengine. The turbocharger's lubrication system is specially designed to copewith high temperatures.
The oil drain pipe is of large diameter to ensure that the oil, whichdevelops a creamy consistency after going through the turbocharger, will drainback to the sump under gravity. If there were a restricted flow in this pipe,it would cause a build-up of pressure around the bearing in the centre housingthat would result in oil leaks on the turbocharger.
Some turbos have a water-cooled centre bearing to reduce heat still further.The advantage is that, because the water is still being warmed by the engine,it continues to circulate and take heat away from the bearing for a few minutesafter the engine has been stopped.
Early criticisms of turbo engines were their poor performance off-boost -when the engine was not turning fast enough to spin the turbine quickly - andthe amount of time it took for the turbocharger to start boosting once theaccelerator was pressed.
The poor off-boost performance was because road-going turbo engines do notusually have a very high compression ratio. Forcing a lot of pressure into thecylinders is equivalent to raising the compressionratio so, if the enginestarted with high compression, at high boost the pressures inside the enginecould promote detonation problems, or 'knock', which would result in seriousengine damage.
As a rough guide, every three pounds of boost are equivalent to increasingthe compression ratio by a factor of one. So if an engine with a compressionratio of 8:1 had a turbo which could deliver nine pounds of boost, theeffective compression ratio would be about 11:1. An average family car has acompression ratio of 9:1.
Better engine and turbo control is the answer - almost all turbo systems nowuse some form of engine management which looks after the electronic ignitionand fuel injection systems, retarding the ignition slightly if the enginestarts to knock. Saab's APC (Automatic
Performance Control) system goes one step further. Not only does it reduceboost pressure to a safe level, it also allows the engine to be run on anygrade of fuel because the management system automatically compensates -although you get the best performance only with the highest grade.
Early turbo engines suffered turbo lag, partly through poor enginemanagement and partly because the lack of suitable turbo units often meant thatthe engines and turbos were not ideally matched to each other - a large turboon a small engine will give good top-end power but will lack flexibility. Lagis almost inevitable because a small engine would take time to `spin up' alarge turbo unit. A small turbo on a large engine gives good mid-range powerwith little or no lag, but ultimate power is compromised.
These problems have been minimized by better matching of the turbo andengine sizes, and by using lighter materials such as ceramics and new designssuch as variable flow nozzles (see sideline overleaf).
The obvious benefit from a turbocharged engine is that of increasedperformance combined with economy - a turbocharged two-litre engine givessimilar performance to an unturbocharged three-litre one, without burning muchmore fuel than a two-litre.
It's often simpler for a manufacturer to turbocharge an existing engine thanto design and develop a new, larger one. Adding a turbo to an engine does notusually significantly increase fuel consumption unless the enhanced performanceis used to the full.
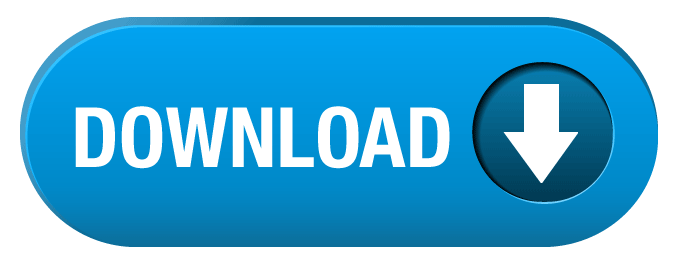